Introduction: Lattice structures show good potential for the design and fabrication of porous orthopedic implants. Indeed, porous structures have lower stiffness than their bulk counterparts which can reduce stress shielding and improve their mechanical compatibility with bones. This compatibility can be enhanced further if the pore size is locally adjusted in conformity with specific load-bearing and bone ingrowth requirements[1]. In this work, a design workflow for such porosity-graded lattice structures is described (see Fig. 1).
Methodology: A voxel-based method with a diamond-type unit cell is proposed to model the lattice structure. The first step of the workflow consists in building the implant geometry and in splitting it into a given number of cells via a voxelisation algorithm. Voxels are then filled with unit cells to produce a diamond-type lattice structure[2]. Afterwards, a finite element mesh is created by modeling the struts of the lattice structure with beam elements. Stresses and strains are then calculated to verify if the stiffness and resistance requirements are met. If any of these two requirements is not respected, beam diameters are locally adjusted to reach the required levels of stiffness and stress. Once both requirements are satisfied, an STL file of the structure is generated by creating a tessellated network of truncated tetrahedrons and struts. In areas where beam diameters are modified, the size of the truncated tetrahedron nodes is adjusted accordingly. Lastly, with the generated STL file, components can be fabricated using a metallic additive manufacturing process such as selective laser melting technology.
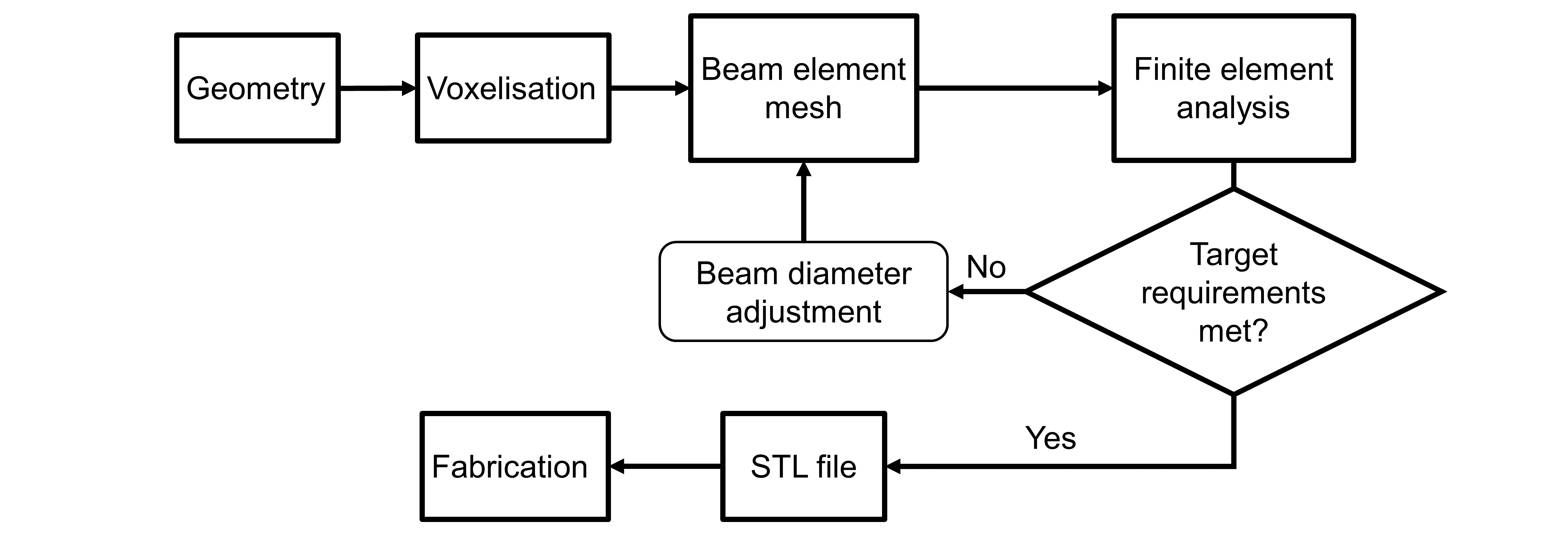
Figure 1: Porosity-graded lattice structure design workflow
Results: The workflow was partially applied for the design of a hip stem implant (Fig. 2). An STL file of the stem geometry was imported into Matlab (Fig. 2a) and voxelised (Fig. 2b). The voxel representation was used to map the nodal positions and connectivity of the diamond-type lattice structure. The map was then imported into ANSYS and a beam finite element mesh was created via its parametric design language (Fig. 2c). At the time of writing, the beam diameters could not be adjusted automatically according to some pre-set stiffness and resistance requirements. Instead, a homogeneous mesh was exported back into Matlab where an STL file of the lattice structure was generated (Fig. 2d).
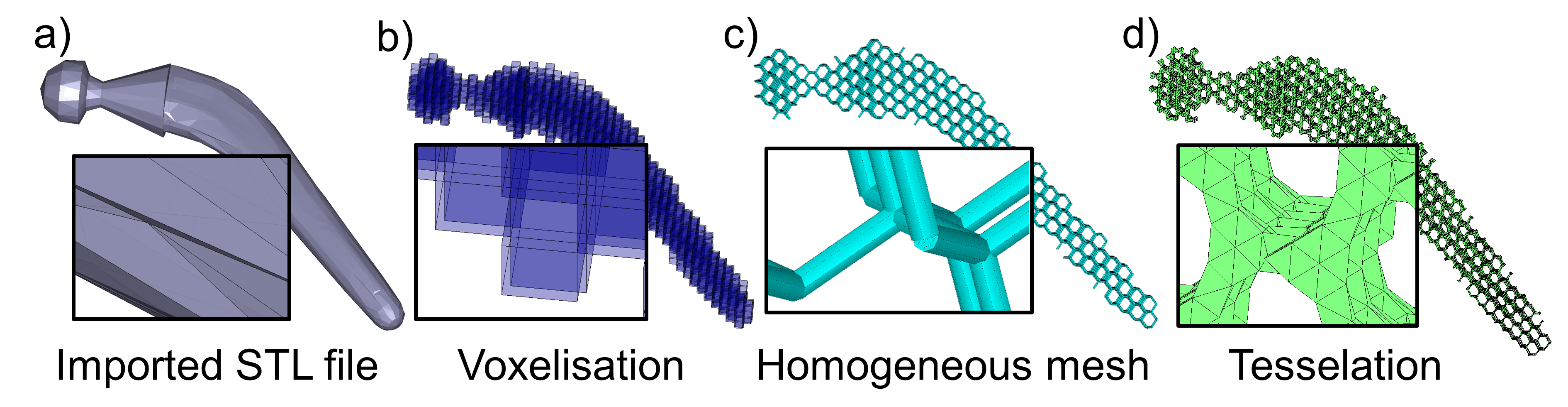
Figure 2: Representations of the workflow steps applied to a hip stem implant
Conclusion: Although the design workflow has been only partially applied, it shows good promise for the design of lattice structures with graded porosity. In future work, automatic beam diameter control will be investigated. Since one of the limitations of this approach is the use of beam elements even for thick struts where the classic beam theory is no longer valid, mechanical tests will be carried out to evaluate the modeling error.
This work is financially supported by the Natural Sciences and Engineering Research Council of Canada (NSERC)
References:
[1] Murr, L. E., et al. "Next-generation biomedical implants using additive manufacturing of complex, cellular and functional mesh arrays." Philosophical Transactions of the Royal Society of London A: Mathematical, Physical and Engineering Sciences 368.1917 (2010): 1999-2032.
[2] Ahmadi, S. M., et al. "Mechanical behavior of regular open-cell porous biomaterials made of diamond lattice unit cells." Journal of the mechanical behavior of biomedical materials 34 (2014): 106-115.