Introduction: Structural bone allografts are a viable option in reconstructing massive bone defects in patients following musculoskeletal (MSK) tumour resection and revision hip/knee replacements[3],[6]. To decrease infection risk, bone allografts are often sterilized with γ-irradiation, which consequently degrades the bone collagen connectivity and makes the bone brittle[5],[10]. Clinically, irradiated bone allografts fracture at rates twice that of fresh non-irradiated allografts[9]. Our lab has developed a method that protects the bone collagen connectivity through ribose pre-treatment while still undergoing γ-irradiation. Biomechanical testing of bone pretreated with our method provided 60-70% protection of toughness and 100% protection of strength otherwise lost with conventional irradiation[11]. This study aimed to determine if the ribose-treated bone allografts are biocompatible with host bone.
Methods: The New Zealand White rabbit (NZWr) radius segmental defect model[1] was used, in which 15-mm critically-sized defects were created. Ethics approval was obtained. Bone allografts were first harvested from the radial diaphysis of donor female NZWr, and treated to create 3 graft types: C=untreated controls, I=conventionally-irradiated (33 kGy), R=our ribose pretreated + γ-irradiation method[11]. Recipient female NZWr (n=24) were then evenly randomized into the 3 graft groups. Allografts were surgically fixed with a 0.8-mm Kirschner wire. Post-operatively, X-rays were taken at 2, 6, and 12 weeks, with bony healing assessed by an independent blinded MSK radiologist using an established radiographic scoring system[1]. The reconstructed radii were retrieved at 12 weeks and analyzed using static bone histomorphometry and μCT. Kruskal-Wallis and Mann-Whitney tests were utilized to compare groups, with statistical significance when p<0.05.
Results: Radiographic analysis revealed no differences in periosteal reaction and degree of osteotomy site union between the groups at any time point (Figure 1). Less cortical remodeling was observed in R and I grafts compared to untreated controls at 6 weeks (p=0.004), but was no longer evident by 12 weeks. Radiographic union was achieved in all three groups by 12 weeks. Histologic and μCT analysis further confirmed union at the graft-host bone interface, with the presence of mineralizing callus and osteoid (Figure 2).
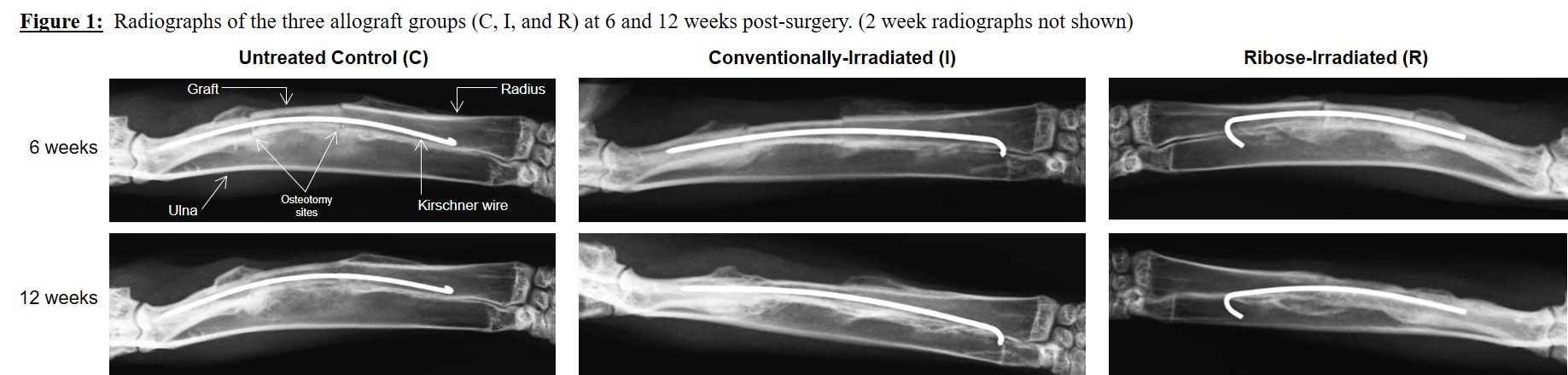
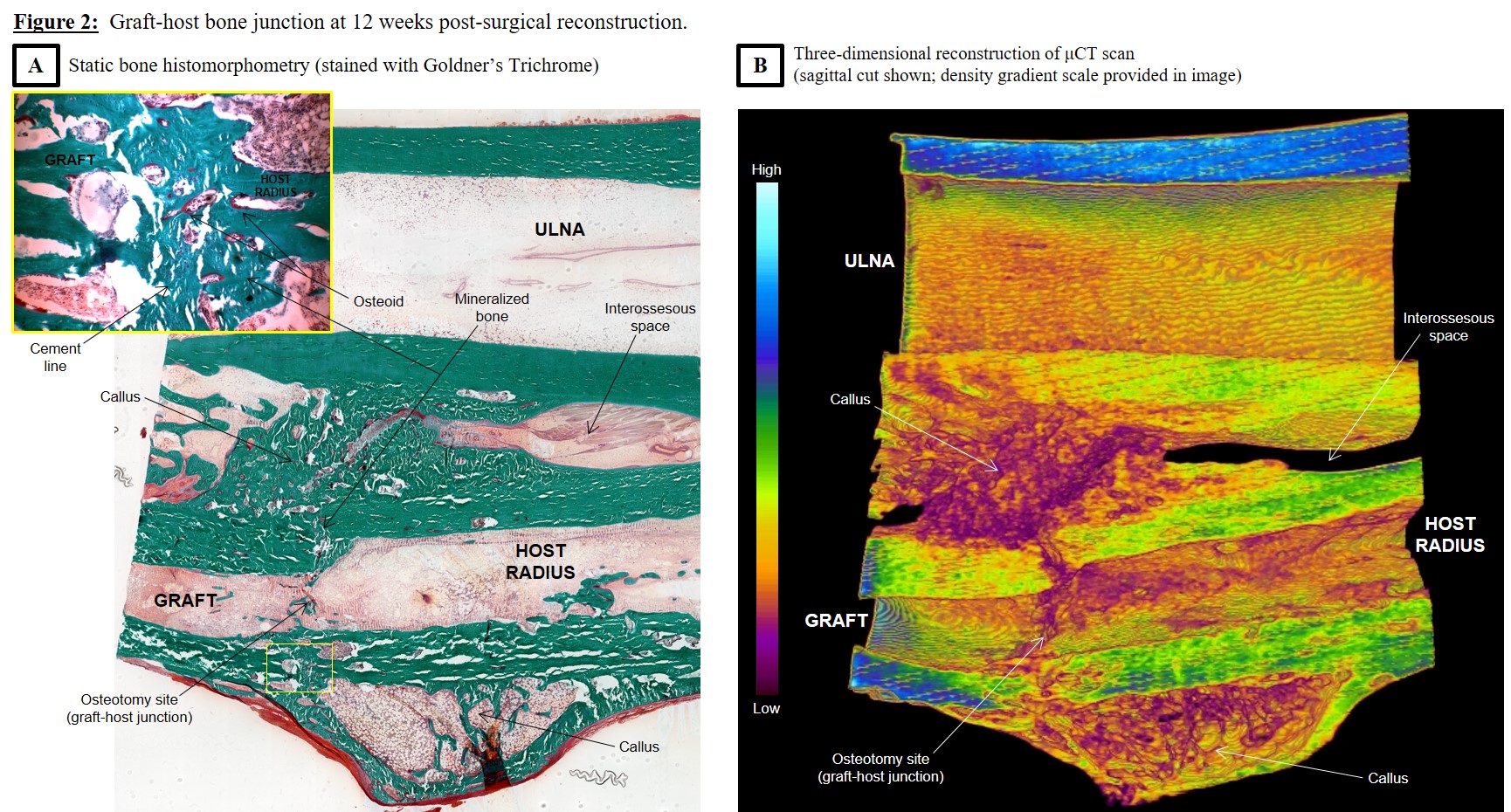
Discussion: Previous studies have shown that the presence of non-enzymatic glycation end products in bone can impair fracture healing[4],[8]. However, these studies investigated bony healing in the setting of diabetic states. Our findings showed that under normal conditions, ribose-irradiated grafts healed at rates similar to controls. Histomorphometry also showed the union of ribose-treated grafts with host bone exhibited similar histological findings (bridging external callus originating from host bone periosteum, distinct cement line between allograft and host bone) as retrieved human allografts clinically in use[2],[7].
Conclusion: Our findings that ribose pretreated irradiation-sterilized bone allografts are biocompatible with host bone in the rabbit helps to further advance this technology for clinical trials. Potential future clinical applications of ribose-treated allografts in orthopaedic patients could help to reduce allograft fracture rates and the need for subsequent revision surgery.
Rainer de Guzman (University of Toronto, Division of Comparative Medicine); Arin Dunning (University of Toronto, Division of Comparative Medicine); Dezan Rego (Mount Sinai Hospital, Surgical Skills Centre)
References:
[1] An Y, Friedman R. Animal models of bone defect repair. In: An Y, Friedman R, eds. Animal Models in Orthopaedic Research. 1st ed. Boca Raton: CRC Press; 1999:241-260.
[2] Bauer T, Muschler G. Bone graft materials. An overview of the basic science. Clin Orthop Relat Res. 2000;371:10-27.
[3] Blackley H, Davis A, Hutchison C, Gross A. Proximal femoral allografts for reconstruction of bone stock in revision arthroplasty of the hip: A nine to fifteen-year follow-up. J Bone Joint Surg Am. 2001;83(3):346-354.
[4] Blakytny R, Spraul M, Jude E. Review: The diabetic bone: a cellular and molecular perspective. Int J Low Extrem Wounds. 2011;10(1):16-32.
[5] Burton B, Gaspar A, Josey D, Tupy J, Grynpas M, Willett T. Bone embrittlement and collagen modifications due to high-dose gamma-irradiation sterilization. Bone. 2014;61:71-81.
[6] Donati D, Di Liddo M, Zavatta M, et al. Massive bone allograft reconstruction in high-grade osteosarcoma. Clin Orthop Relat Res. 2000;377(Aug):186-194.
[7] Enneking W, Campanacci D. Retrieved human allografts: a clinicopathological study. J Bone Joint Surg Am. 2001;83-A(7):971-986.
[8] Hamada Y, Kitazawa S, Kitazawa R, Fujii H, Kasuga M, Fukagawa M. Histomorphometric analysis of diabetic osteopenia in streptozotocin-induced diabetic mice: A possible role of oxidative stress. Bone. 2007;40(5):1408-1414.
[9] Lietman S, Tomford W, Gebhardt M, Springfield D, Mankin H. Complications of irradiated allografts in Orthopaedic tumor surgery. Clin Orthop Relat Res. 2000;375(June):213-217.
[10] Nguyen H, Morgan D, Forwood M. Sterilization of allograft bone: effects of gamma irradiation on allograft biology and biomechanics. Cell Tissue Bank. 2007;8(2):93-105.
[11] Willett T, Burton B, Woodside M, Wang Z, Gaspar A, Attia T. γ-Irradiation sterilized bone strengthened and toughened by ribose pre-treatment. J Mech Behav Biomed Mater. 2015;44C:147-155.