Introduction: Materials intended as tissue engineering scaffolds must be porous to accommodate cells and allow fluid flow for the transport of nutrients and removal of waste products. These are both necessary for long term cell viability[1]. The permeability of materials to fluid flow affects not only the biophysical stimuli received by cells seeded in the scaffold: the pressure and stress exerted by the fluid on such cells and the scaffold’s structure also depend on the permeability.
Hydrogels and freeze-dried constructs are commonly investigated as cell scaffolds, as one can control their porosity and consequent water content to achieve suitable conditions for cell seeding. However, the permeability of such materials is poorly understood as a result of their multi-scale structure: available models are too simplistic to describe the interaction between the fluid and the material making up the nano- or microporous architecture. The present work attempts to find generalized relationships to model the fluid permeability as a result of the multi-scale geometry of the structures.
Materials and Methods: Freeze-dried type I collagen sponges[2] were fabricated using varying solid concentrations between 0.5 % w/V and 1.5 % w/V. Their morphology was assessed by X-ray Micro-Computed Tomography (micro-CT). Micro-structured, responsive hydrogels were prepared by freeze-thawing 15 % w/w solutions of poly(vinyl alcohol) (PVA) and poly(acrylic acid) (PAA) for a varying number of cycles. Their microstructure in the swollen and not-swollen states was investigated by confocal microscopy.
The permeability of the materials fabricated was quantified for the bulk structures through a custom-built device for measuring permeability based on differential fluid pressure. The local, multi-scale permeability of the materials was assessed by indentation on an Instron 5544 universal testing machine, utilizing a poroelastic analysis framework[3]. The results were used to understand and model the permeability as a result of hierarchical structure parameters, such as porosity and interconnectivity.
Results and Discussion: The morphology of the freeze-dried collagen scaffolds showed a constant pore size with increasing concentration of solid. However, the pores became gradually more closed as the pore wall coverage increased from 29 % to 65 %. As a result, the permeability of the structure decreased ten-fold with increasing concentration (Figure 1), varying with porosity and interconnectivity rather than pore size.
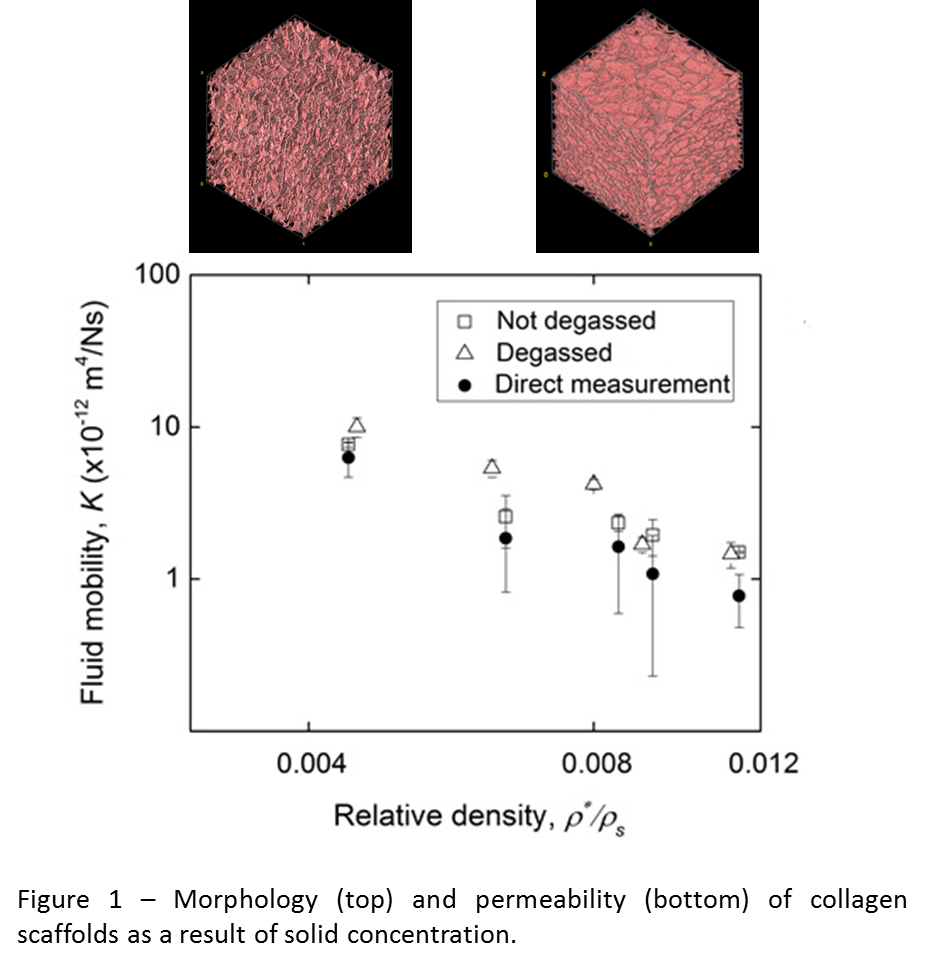
A similar relationship was found for the bulk permeability of responsive hydrogels: the swollen structure showed a larger pore size but a similar micro-porosity (~40 %), therefore the permeability did not change upon swelling. However, the nano-porosity (Figure 2) of the gel making up the structure (~99 %) increased with swelling, and so did its permeability as a result.
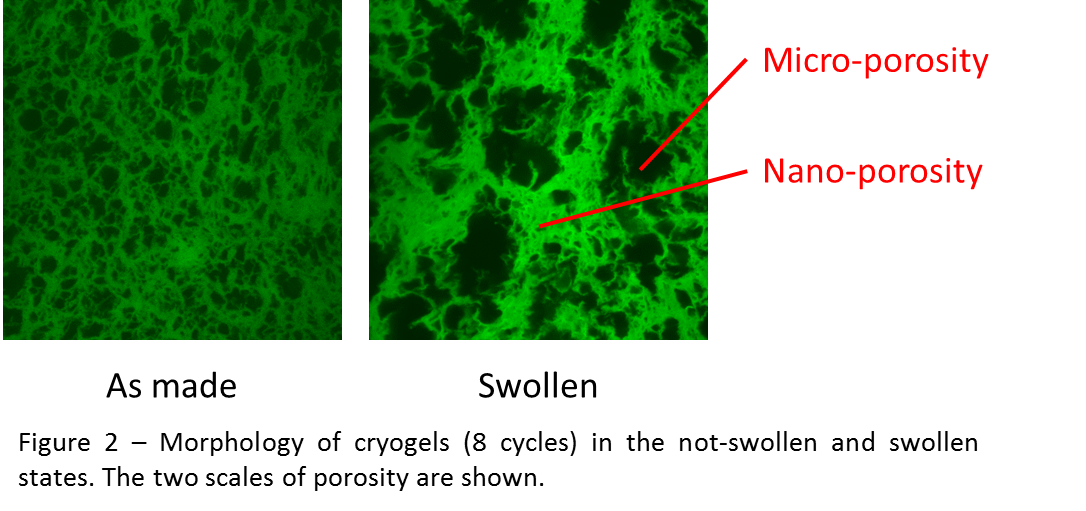
Conclusion: The fluid permeability of various scaffolds was found to depend on structural parameters such as porosity and interconnectivity, but not on pore size. The magnitude of permeability can vary at the nanoscale while remaining constant at the microscale, and is dependent on the mechanical response of the material. These results provide general relationships that can be applied to quantify the permeability of other tissue engineering scaffolds.
Nano Doctoral Training Centre (NanoDTC); EPSRC; Ruth E. Cameron; Jennifer C. Ashworth
References:
[1] F.J. O'Brien et al., “The effect of pore size on permeability and cell attachment in collagen scaffolds for tissue engineering.” Technology and Health Care (2007): 15, 3.
[2] G.S. Offeddu et al., “Multi-scale mechanical response of freeze-dried collagen scaffolds for tissue engineering applications”. J. Mech. Behav. Bio. Mats. (2015): 42, 19.
[3] M.L. Oyen, “Spherical indentation creep following ramp loading.” J Mater Res (2005), 20: 2094.